Time-resolved dose rate measurements in pencil beam scanning proton FLASH therapy
OC-0281
Abstract
Time-resolved dose rate measurements in pencil beam scanning proton FLASH therapy
Authors: Eleni Kanouta1, Per Poulsen1, Gustavo Kertzscher1, Mateusz Sitarz1, Brita Sørensen1,2, Jacob Johansen1
1Aarhus University Hospital, Danish Centre for Particle Therapy, Aarhus, Denmark; 2Aarhus University Hospital, Department of Experimental Clinical Oncology, Aarhus, Denmark
Show Affiliations
Hide Affiliations
Purpose or Objective
Dose rate
verification in FLASH radiotherapy is essential for validating the dosimetric
conditions that trigger the FLASH effect. Dose rate validation with good time
resolution is particularly important for pencil beam scanning (PBS) where the
instantaneous dose rate in a point varies on a millisecond time scale as the
beam scans across the spot pattern. In this study, a scintillator-based
detector system was used for time-resolved dose rate measurements in proton PBS
irradiations. The dose rate response of the system was characterized, and the
calibrated system was used to measure the instantaneous dose rate in pre-clinical
proton FLASH studies with mice.
Material and Methods
The detector
system was based on four sub-millimeter fiber-coupled ZnSe:O scintillators. It measured
the instantaneous dose rate at 50kHz, sufficient to provide the dose rate of
each spot individually. As a first step, the detector system was calibrated. The
detectors were placed on an Advanced Markus ionization chamber (IC) inside a
water bath in the isocenter plane (Fig. 1A). A 52mm-by-52mm spot pattern with
2mm spot spacing was delivered using a 250MeV proton beam (Fig. 1B). The dose
rate was altered by changing the requested nozzle beam current from 5nA to
215nA with 10nA steps. A calibration curve (Fig. 1C) was produced by plotting
the mean detector signal [V] for each spot and nozzle current against an
absolute dose rate calculated from the IC measurement [Gy/s]. The signal and
instantaneous dose rate depended on the distance to the spot and the beam
current. The integral dose from the IC was recalculated into a dose rate per
spot by assuming each spot contributed to the total dose by a weight depending
on the detector-to-spot distance and the spot duration. Moreover, the signal
was used for locating the detector positions within the spot pattern and
measuring the spot widths. The calibration was then applied to in vivo
measurements during mouse experiments, in order to provide the instantaneous
dose rate. The measured dose rates were compared to the dose rate determined
from the spot size and the MU-rate found in machine log files, which was
assumed to be the ground truth.
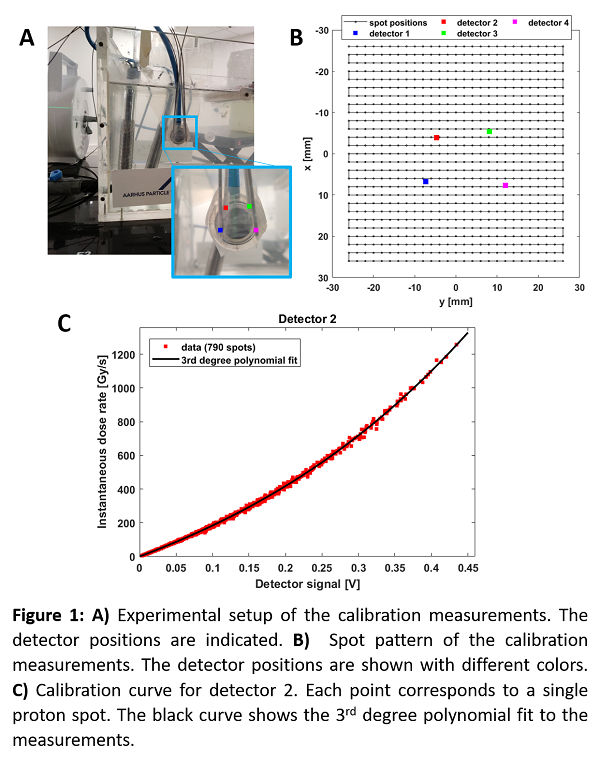
Results
For each detector, the instantaneous dose rate as function of measured
detector signal was modelled with a third-degree polynomial function (Fig. 1C).
The calibrated system enabled instantaneous dose rate measurements for each
delivered spot during mouse irradiations (Fig. 2). This dose rate was in good
agreement with the ground truth dose rate with a root-mean-square difference of
13Gy/s across a wide range of instantaneous dose rates up to 1000Gy/s (Fig. 2B).
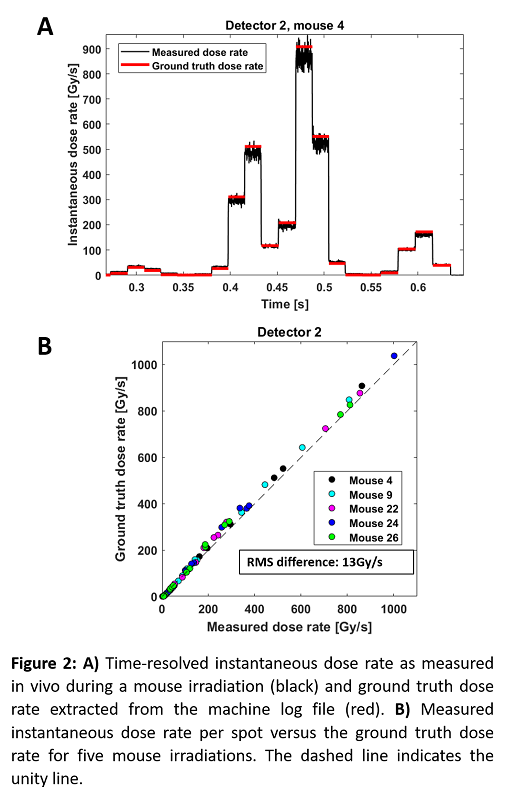
Conclusion
A detector
system for direct measurements of the instantaneous dose rate was developed and
calibrated in a wide range of dose rates. The detector system was successfully
applied in vivo in mouse irradiations, enabling time-resolved dose rate
measurements in pre-clinical proton FLASH studies on a sub-spot level.