Assessment of biology-guided radiotherapy (BgRT) Accuracy using Emulated delivery technique
Oluwaseyi M. Oderinde,
USA
PO-1660
Abstract
Assessment of biology-guided radiotherapy (BgRT) Accuracy using Emulated delivery technique
Authors: Siddique Khan1, Murat Surucu2, Manoj Narayanan3, Maksat Haytmyradov3, Shiyu Xu3, Peter Olcott3, Yevgen Voronenko3, Oluwaseyi M. Oderinde4,5, Gopinath Kuduvalli3, Shervin M. Shirvani6
1RefleXIon Medical, Inc, R&D, Hayward, USA; 2Stanford University, Department of Radiation Oncology, Palo Auto, USA; 3RefleXion Medical, Inc , R&D, Hayward, USA; 4RefleXion Medical, Clinical, Hayward, USA; 5RefleXion Medical, Inc , Clinical, Hayward, USA; 6RefleXion Medical, Inc, Clinical, Hayward, USA
Show Affiliations
Hide Affiliations
Purpose or Objective
New techniques in radiotherapy respond to intra-treatment information to generate machine instructions “dynamically determined during delivery (D4)”. One example is biology-guided radiotherapy (BgRT), an investigational technique that utilizes packets of positron emission tomography (PET) signal that emanate outward from a malignant tumor to direct beamlets of radiotherapy back to the target with subsecond latency. For these novel systems, machine instructions for the linear accelerator (LINAC) and multileaf collimator (MLC) are different for every fraction. Therefore, new forms of physical delivery validation may be needed to complement established physics quality assurance (QA) methods because the latter assume unchanging machine instructions at each fraction. Herein an investigational methodology, “emulated delivery”, is described and physically validated for assessing “D4” system
Material and Methods
BgRT plans directed at 18F-Fluorodeoxyglucose (FDG) phantoms were used to investigate emulated delivery. BgRT treatment plans were created, approved, and quality assured using imaging-only PET acquisitions for both a static and a moving (sinusoidal motion of 2 cm amplitude and 4 s/cycle using CIRS motion platform) FDG phantom. For both experiments, the 22mm diameter spherical target and the background were filled with FDG to achieve a target-background ratio of approximately 8:1. To emulate BgRT treatment delivery, a short duration pre-scan PET image (to confirm stable PET at the delivery timepoint) and a longer duration PET scan were acquired. The PET emission data from the longer scan was binned into limited time-sample packets and converted to partial fluences using the BgRT algorithm. Those fluences were then modeled onto a digital model of the FDG phantom in silico to generate the resultant dose distribution and then physically delivered into QA phantoms. A points-within-bounded-DVH (pwbDVH) metric was used to assess the accuracy of the on-the-fly dose distributions, and 3D Gamma passing criteria (3%/3mm) was used to assess physical deliverability.
Results
BgRT plans were created successfully and passed the initial PET evaluation during delivery of the treatment fraction. The emulated BgRT delivery achieved a pwbDVH and gamma pass rate of 100% and 99.88% for the static FDG target, and 100% and 99.88% for the moving FDG target.
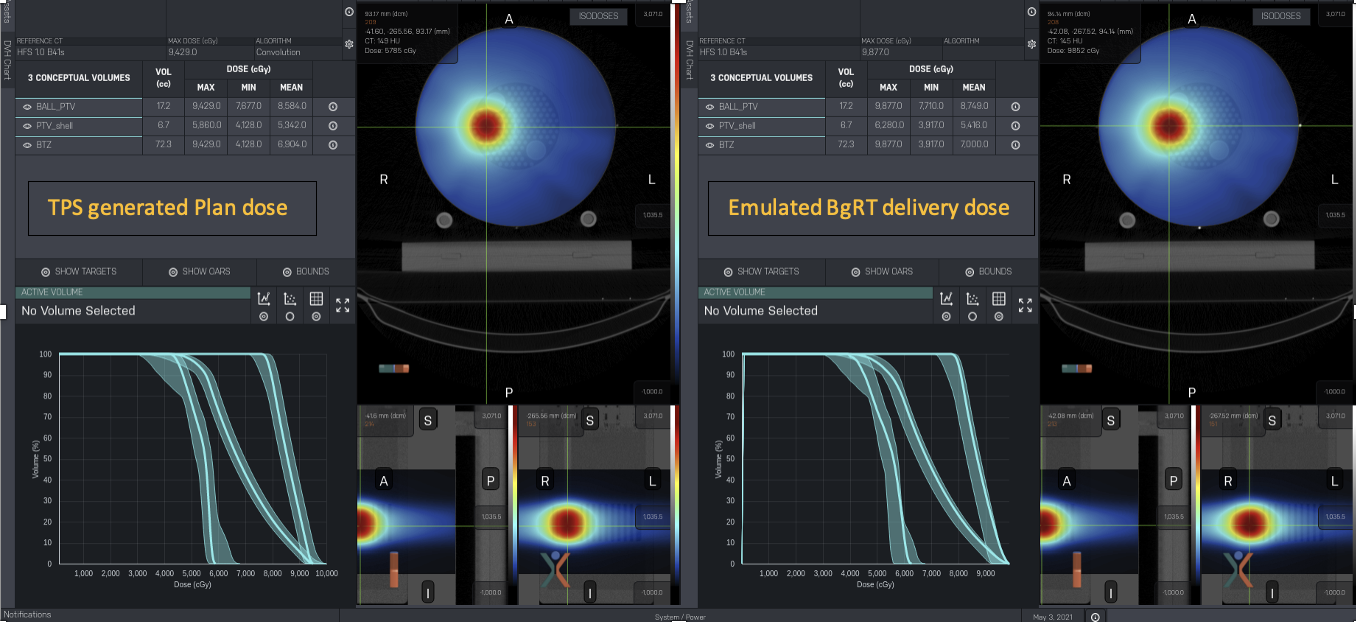
Conclusion
Emulated delivery provides a coherent and reproducible methodology for assessing dose accuracy in novel systems that employ signal-response feedback loops to calculate and deliver “on-the-fly” radiotherapy plans. In this experiment using FDG phantoms, the emulated delivery methodology confirmed accurate and deliverable BgRT dose distributions.