Advanced 3D printing for heterogeneous and dynamic phantoms for dosimetry and imaging
Gabriel Paiva Fonseca,
The Netherlands
PO-1640
Abstract
Advanced 3D printing for heterogeneous and dynamic phantoms for dosimetry and imaging
Authors: Gabriel Paiva Fonseca1, Murillo Bellezzo2, Robert Voncken3, Behzad Rezaeifar4, Teun van Wagenberg4, Niklas Lackner4, Frank Verhaegen4
1Maastricht University, Radiotherapy, Maastricht, The Netherlands; 2Maastricht university, radiotherapy, Maastricht, The Netherlands; 3Maastro, radiotherapy, Maastricht, The Netherlands; 4Maastricht University, radiotherapy, Maastricht, The Netherlands
Show Affiliations
Hide Affiliations
Purpose or Objective
Technological
developments in 3D printing resulted in applications in several fields
including radiotherapy. It brings the opportunity to research and clinical
departments to develop new technologies and prototypes reaching the same level
of accuracy as commercial vendors. This report describes some of the
applications of 3D printing in our clinic and focuses on the 3D
printing technology rather than the detailed description of each application.
Material and Methods
Commercial and
in-house developed 3D printers were introduced in our research department early
in 2018 aiming to manufacture and test an in-house developed brachytherapy
applicator for rectal cancer and develop a new device for applicator
commissioning. This technology was then employed to develop custom 3D printed homogeneous
and heterogeneous phantoms based on patient anatomy for dosimetry and imaging and
also to develop prototypes of motion platforms currently being used in
combination with 3D printed phantoms for dosimetry. Although great geometrical
accuracy can be obtained, the material properties are often not well known. In
this study, more than 20 types of commercial materials (e.g., PC, PLA, ABS, PP,
Nylon, and TPU) were tested. In addition, a custom filament including ≈17% of Ca was developed to mimic bone.
Results
Fast prototyping
using 3D printing technologies was essential to quickly design and manufacture
applicators, phantoms and other devices with limited costs. The brachytherapy
applicator (Figure 1a) was eventually patented and further developed into a
commercial product by a major radiotherapy device vendor whilst the applicator
commissioning device (Figure 1b) is currently under clinical implementation.
Treatment verification methods are currently being developed using prostate
(Figure 1c) and head phantoms (Figure 1d) to perform static and dynamic
measurements using the 3D printed motion platform (Figure 1d). A phantom
(Figure 1e) based on the anatomy of a large patient (developed due to the lack
of commercial alternatives) was used to evaluate extended field-of-view CT
reconstruction. Tissue-equivalent materials for photon and proton therapy are
desirable for imaging and dosimetry with bone equivalent materials being the main
challenge. Our custom bone material (Figure 2a) showed similar behaviour as
commercial tissue-mimicking inserts regarding density, Hounsfield Units, and stopping
power ratios. In addition, mass attenuation coefficients are similar to ones
obtained using bone composition described in the literature.
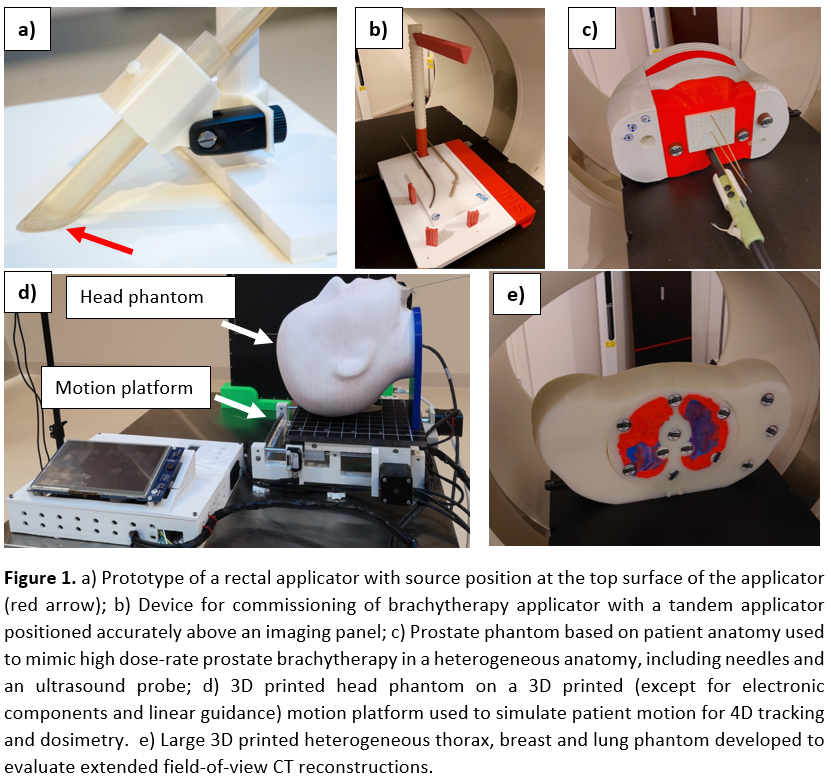
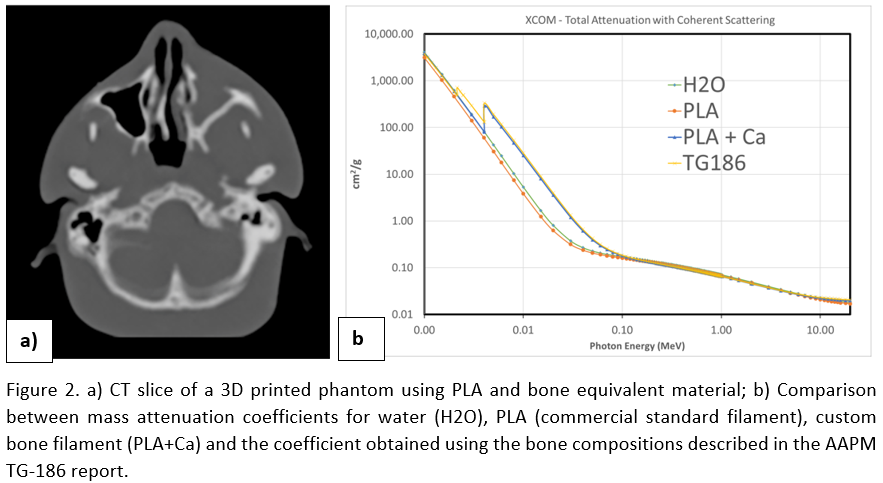
Conclusion
3D printing
applications in radiotherapy or by specialized companies will continue to
increase providing an essential tool for affordable innovation and
customization (e.g. patient or application-specific phantoms). Nonetheless, in-house
developed prototypes need medical certification through regulations, risk
analysis, documentation and other requirements for implementation for which
hospitals may not be prepared.