An empirical approach for metal implant classification using 2D dual energy radiography
PO-1624
Abstract
An empirical approach for metal implant classification using 2D dual energy radiography
Authors: Jens Edmund1,2, Ulf Bjelkengren1
1Gentofte and Herlev Hospital, University of Copenhagen, Radiotherapy Research Unit, Department of Oncology, 2730 Herlev, Denmark; 2Niels Bohr Institute , University of Copenhagen, 2100 Copenhagen, Denmark
Show Affiliations
Hide Affiliations
Purpose or Objective
The
composition of metal implants (MI) is often unknown, leading to wrongful
material assignments in radiotherapy (RT) dose planning. CT numbers of MI can
be ambiguous due to the combined effects of photoelectric and Compton
interactions at low scanning energies (keV) and the high charge (Z) of metals.
Further, CT numbers can be incorrect due to beam hardening and photon
starvation modelling effects. To address these concerns, we investigate whether
2D dual energy radiographs, simulating 2D CT topogram projections, can provide
a bulk effective charge (Zeff) classification of MI.
Material and Methods
We included
bone and metals inserts (Cirs and Gammex Inc.) with known charges for modelling
covering Z/Zeff=10 (Inner bone) to 29 (Copper). Zeff=√(∑iwiZi2.98)1/2.98
where wi is the fraction of total electrons of material i. 4 unknown
materials were evaluated; the head of a hip implant (HipHead) and proximal
femoral rod (rod) from Corail Hip® systems, teeth with dental implants
(teeth) and a stainless steel alloy (SS_unknw) insert (Gammex Inc.).
Radiographs
with (I) and without (I0) materials were obtained using the on-board
imaging system on a Varian TrueBeam v2.7 (Varian Medical Systems) at energies
(E)= 70, 80, 100, 120 and 140kV and constant mAs=0.5. Radiographs E>80kV
were also acquired with a Ti filter (ETi). Projections p(E)=ln[I0(E)/I(E)]
were generated and ratios pR=p(EHigh)/p(ELow) were
created where EHigh are all E>ELow. Average pR values
were extracted for each material.
A
mono exponential empirical model Zeff=exp[a0+a1·pR] was fitted for each dual energy pair to
search for an optimal combination. Further, materials with a larger Z and a
quadratic pR term were investigated but did not improve the model predictions. Thus
a simpler model in a more relevant Z interval was chosen. p(E) and a0
values < 0 were disregarded as too noisy (I>I0) or non-physical
(Z<1). Only E combinations proving a reasonable fit (R2>0.8) were
used for Zeff prediction.
Results
pR
with combinations ELow-(EHigh) =70-(100/120/120Ti/140Ti),
80-(100/120/120Ti/140/140Ti), 100-(140/140Ti),
100Ti-(120/140/140Ti), 120Ti-(140/140Ti)
kV all had similar fits (see figure).
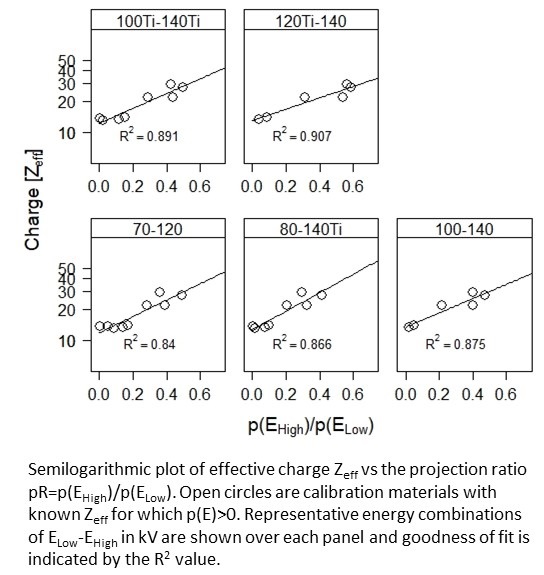
The corresponding estimated Zeff
intervals were 22-24 for rod (Ti+SS alloys, Z:20-30), 21-27 for teeth (bone+Au+Sn+Cu,
Z:14-45), 28-36 for SS_unknw (Cr+F+Ni+Cu+Mo, Z:24-42) and 30-41 for HipHead (Ti-Al+Co/Cr
alloys, Z<27) ±
15-48% range uncertainty. Known model materials could be predicted with 4-21%
uncertainty (see table). All estimated Zeff values of the unknown
materials were within a theoretical Z interval except for HipHead which was estimated
higher but within the uncertainty range.
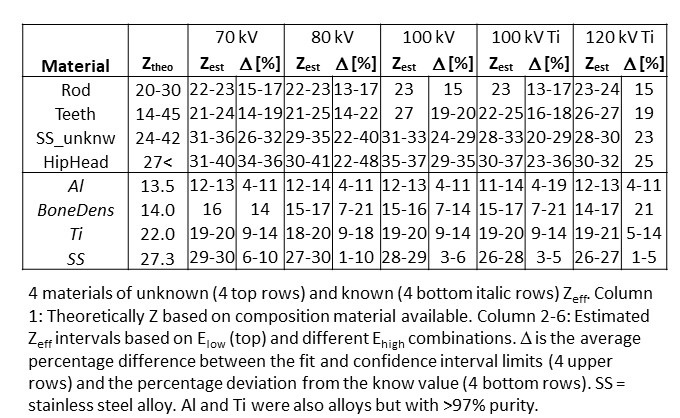
Conclusion
This method
provides a first step towards allocating MI to material specific dose kernel by
estimating their Zeff from dual energy topograms. Increased mAs
impact on imaging plate gain factors for uncertainty reduction and adding MI in
anthropomorphic phantoms should be further investigated.