A novel system and approach for proton radiography using a monolithic scintillator detector
PO-1592
Abstract
A novel system and approach for proton radiography using a monolithic scintillator detector
Authors: Chinmay Darne1, Daniel Robertson2, Charles-Antoine Collins-Fekete3, Sam Beddar1
1The University of Texas MD Anderson Cancer Center, Radiation Physics, Houston, USA; 2Mayo Clinic Arizona, Radiation Oncology, Phoenix, USA; 3University College London, Medical Physics and Biomedical Engineering, London, United Kingdom
Show Affiliations
Hide Affiliations
Purpose or Objective
In this study, we demonstrate a novel system
design for proton radiography and use two imaging approaches to generate proton
radiographs. The novel system design consists of a monolithic scintillator detector
which captures complete proton beam deposition and circumvents the need to
modulate proton beams. Proton radiographs are generated using
projections from 2 cameras: (a) by integrating light along the beam axis
(beam-integration method), and (b) by recording changes to the proton Bragg
peak (BP) location for the beam as it travels through the phantom (percentage
depth light or PDL method).
Material and Methods
The imaging system consists of a monolithic
plastic scintillator detector and two CCD cameras for imaging the scintillation
light distribution (refer Figure 1). A 45° angled mirror redirects light to
camera 2 without directly exposing it to ionizing radiation. Camera 2 generates
images by integrating light along the beam axis. The light
intensity is converted into water equivalent thickness (WET) by plotting an
energy-specific calibration graph. A polynomial fit to this graph is
used to calculate phantom WETs. Camera 1 images BP locations of scanned pencil
beams. The radiograph
is reconstructed by comparing the PDL profiles for these beams that pass through the
phantom with pristine PDL profiles (without phantom) shifted by a convolution
of the WET. A curvelet minimization method is applied to improve image resolution.
Gammex phantoms (solid water, cortical bone) were imaged using the
system (refer Figure 1). The relative percentage accuracy, (WETexpt – WETcalc) / WETcalc
* 100, was
used to evaluate the system’s performance in retrieving WET values for phantoms.
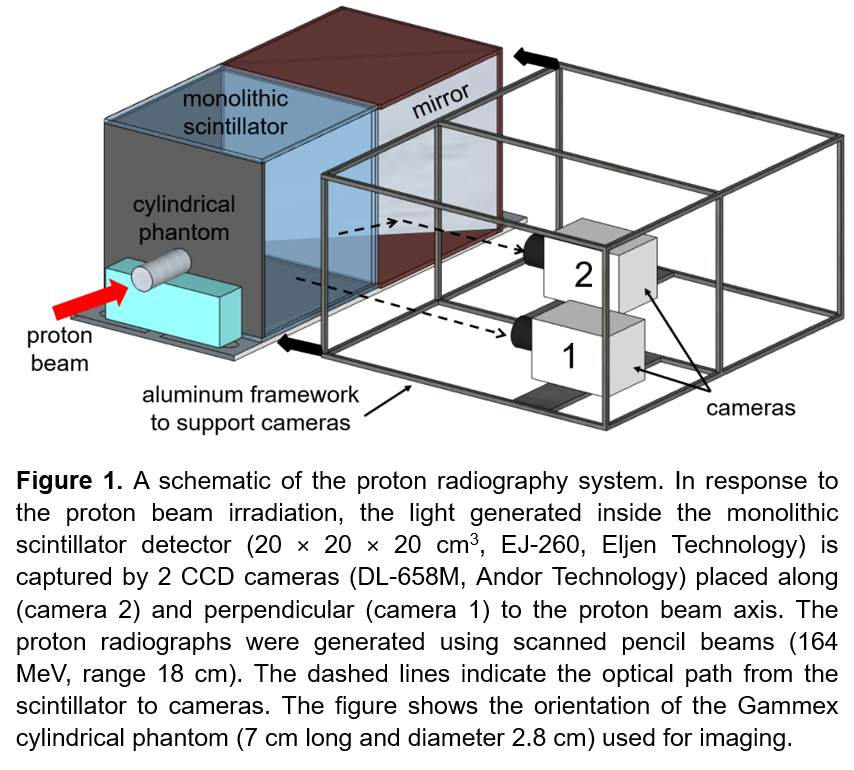
Results
The system characterization studies included
assessing its linearity (R2 = 1) over two orders of magnitude change
in proton dose, camera resolution (0.44 mm/pixel), and short (0.37%) and medium
term (2% over 14 weeks) stability. Figure 2 shows proton radiographs for cortical
bone generated using the two reconstruction methods. The resolution for the
beam-integration method is limited by the CCD sensor pixel size. Blurring at
edges is due to proton scatter occurring within the phantom as well as in the
scintillator volume. The spatial resolution of the PDL method is limited by the
pencil beam size and the image therefore looks pixelated. Relative percentage
accuracies of −0.18 ± 0.35% and −2.94 ± 1.20% for solid water and cortical bone,
respectively, were obtained from the beam-integration method, while accuracies
of −0.29 ± 3.11% and −0.75 ± 6.11% for solid water and cortical bone were calculated
for the PDL method.
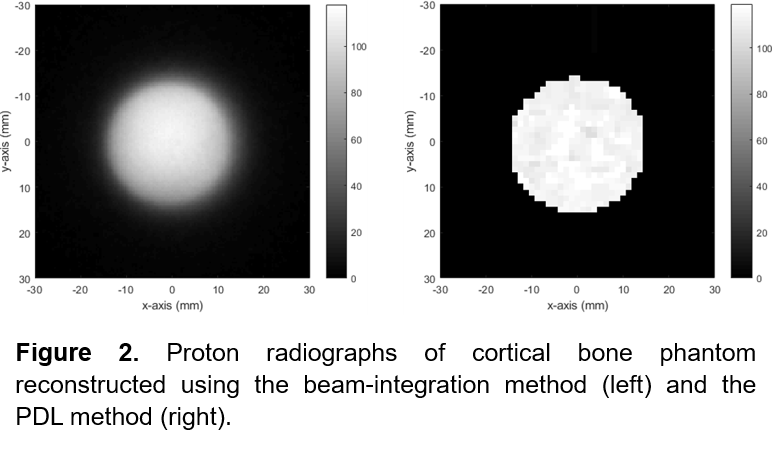
Conclusion
This work suggests that the monolithic
scintillator-based detector system design has the versatility to generate
proton radiographs using two unique imaging methods and with good WET accuracy.
It therefore has the potential to be translated into clinics for treatment
planning and patient alignment for proton radiotherapy.