Development of deformable 3D anthropomorphic dosimetry systems for proton therapy
PO-1572
Abstract
Development of deformable 3D anthropomorphic dosimetry systems for proton therapy
Authors: Simon Jensen1, Tobias Erichsen2, Morten Jensen1, Peter Balling3, Jørgen Petersen4, Per Poulsen1, Ludvig Muren1
1Aarhus University, Department of Clinical Medicine, Aarhus, Denmark; 2Aarhus University, Deparment of Engineering, Aarhus, Denmark; 3Aarhus University, Department of Physics and Astronomy, Aarhus, Denmark; 4Aarhus University Hospital, Department of Medical Physics, Aarhus, Denmark
Show Affiliations
Hide Affiliations
Purpose or Objective
Pencil
beam scanning (PBS) proton therapy offers individualized conformal dose
distributions. However, intra-fractional motion in the abdomen and thorax is a
major challenge and can severely degrade the dose distribution.
Building up to a clinical protocol for liver tumours in our proton centre, we
are developing an anthropomorphic dosimetry phantom, containing clinically
realistic deformable three-dimensional (3D) dosimetry inserts, onto which complex
deformations can be applied.
The aim of this study was to establish a method for producing high precision
anthropomorphic 3D dosimeters to fit into this phantom.
Material and Methods
The
dosimeter moulds were created using a 3D printed organ as a negative. The shape
of an adult-size liver was divided into three parts to minimize the optical
pathlength through the individual dosimeter parts and 3D printed using a polylactic
acid filament. The parts were sanded down, coated with a layer of primer, and
spray-painted to provide a smooth surface (Figure 1).
A casting basin was made from sculpting clay and filled with silicone moulding
rubber. The 3D print was suspended in the basin and the moulding rubber was
left to cure for 24 hours.
The 3D dosimeters were prepared using transparent silicone kit, chloroform, and
the radiation-sensitive component leucomalachite green, poured into the moulds and
cured for 72 hours prior to irradiation. A specially designed nut was suspended
in the dosimeter during curing for later attachment to the read-out system.
The dosimeters were x-ray computed tomography (CT) scanned and imported to the
treatment planning system (TPS). The treatment plan consisted of six conventional
6 MV photon fields (15 mm x 15 mm) evenly spaced on a 180-degree arc with a
prescribed dose of 5 Gy normalized to 100% in target maximum. A four-degrees-of-freedom
couch correction was performed using a cone-beam CT.
The optical density (OD) of the dosimeters was read-out pre- and
post-irradiation using an optical CT scanner with 1000 projections and reconstructed
using the ordered-subsets-convex total-variation method.
The TPS dose distribution was compared to the experimental dose distributions
using a 3%/3 mm 3D gamma analysis including only voxels above 10% of the
maximum TPS dose.
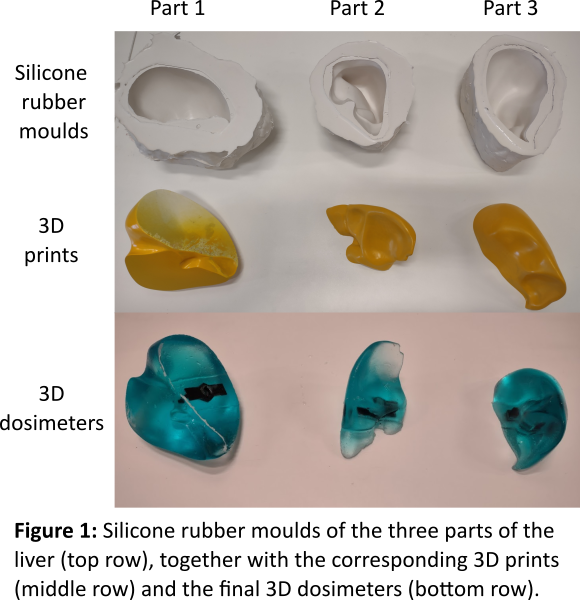
Results
The
silicone rubber moulds were successfully made with great detail reproducing all
visible filament lines from the 3D prints and had the advantage that it was
easy to separate the 3D dosimeters from the moulds compared to rigid moulds. Most
of the dose was delivered in just one of the liver-dosimeter parts (Figure 2) and
here we achieved a gamma pass rate of 87%. The main challenges in the optical
read-out were the dosimeter edges along with the signal-to-noise ratio.
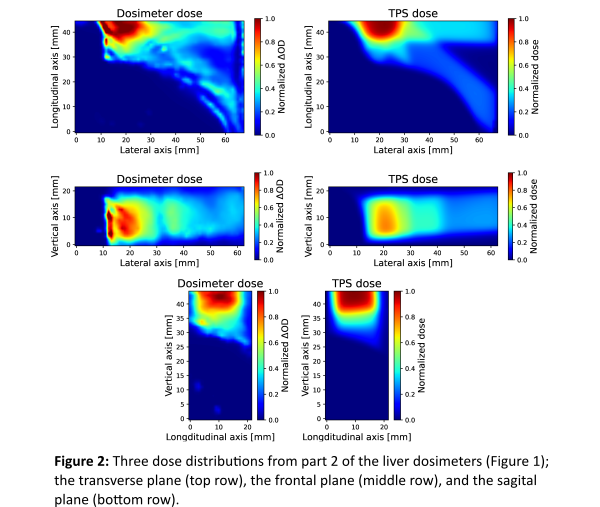
Conclusion
The
described method successfully produced reusable anthropomorphic moulds for 3D
dosimeters. Furthermore, the liver irradiation showed a fair correlation
between the TPS calculation and experimental measurements and indicates its
suitability for future proton irradiations.