Towards MR-guided particle radiotherapy: Compatibility of an open MR scanner with an ion beamline
OC-0778
Abstract
Towards MR-guided particle radiotherapy: Compatibility of an open MR scanner with an ion beamline
Authors: Katharina Paul1,2, Stefan Dorsch3,2, Jakob Naumann4, Thomas Hansmann4, Thomas Haberer4, Jürgen Debus2,1,5,6,7, Sebastian Klüter2,1
1University Hospital Heidelberg, Department of Radiation Oncology, Heidelberg, Germany; 2National Center for Radiation Research in Oncology (NCRO), Heidelberg Institute for Radiation Oncology (HIRO), Heidelberg, Germany; 3German Cancer Research Center (DKFZ), Department of Medical Physics in Radiation Oncology, Heidelberg, Germany; 4Heidelberg Ion-Beam Therapy Center, HIT, Heidelberg, Germany; 5Heidelberg Ion-Beam Therapy Center , HIT, Heidelberg, Germany; 6Clinical Cooperation Unit Radiation Oncology, German Cancer Research Center (DKFZ), Heidelberg, Germany; 7core center Heidelberg, German Cancer Consortium (DKTK), Heidelberg, Germany
Show Affiliations
Hide Affiliations
Purpose or Objective
MR guided
particle radiotherapy holds the potential to increase therapy precision by
daily monitoring of tumour and organ at risk location, size and shape. The ability
to gate the beam to breathing motion has been shown to be
beneficial in MR guided photon therapy (1,2) and is even more important for
particle radiotherapy to ensure precise location of the Bragg peak. Hitherto,
no commercial systems are available, though it has been reported on
experimental set-ups (3). In this work we report on the
installation of an open MR scanner in front of an ion beamline and the analysis
of simultaneous operation of MR imaging and particle irradiation.
Material and Methods
An MR
system consisting of a 0.25 T orthopaedic device featuring a permanent magnet with
a vertical B0 field (Esaote, Italy) within a radiofrequency (RF)-shielded
cabin is positioned in front of a horizontal ion beamline (Fig 1a). The geometrical relationship of MR
and beam isocentre is verified using an external laser system. 12C ions were used due to their higher
mass in comparison to protons. Two sets of experiments were performed: (i)
homogeneous spherical phantom; 3D GRE (TE/TR 14/28ms, spatial res. (0.8x0.8x0.7)mm3); irradiation of a central spot with beam energies from 89MeV to 430MeV;
calculation of the centre of gravity for all slices, SNR
analysis; (ii) geometrical phantom; 2D GRE (TE/TR 10/500ms, spatial res. (0.4x0.4x5)mm3); irradiation of a scanned 2D grid with step size of 5mm; line profile analysis.
Results
Fig. 1c,d
illustrate the range of the x- and y-coordinate of the centre of gravity for
all slices for 5 sequential baseline
measurements and all applied beam energies. The value range of the measurements
with simultaneous spot irradiation is comparable to that of the baseline and the overall range is in the sub-pixel regime, meaning that there is no shift. The
results of the SNR analysis in Fig. 1b show that there is no change in signal
levels when the phantom is irradiated during image acquisition. The line
profiles in Figure 2b covering small structures do not show any offset or shift when comparing the baseline to the acquisition
with active 2D beam scanning.
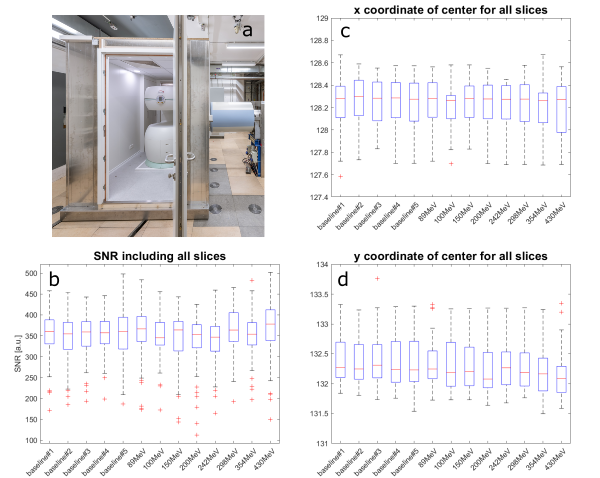
Figure 1: (a)
photograph of the 0.25 T MR scanner in front of the beam line. (b) SNR
values. (c) x-coordinates of the centre of gravity. (d) y-coordinates.
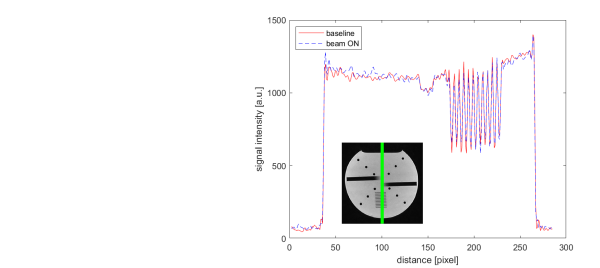
Figure 2:
Line profile along the green line with elements in the sub-millimetre range.
Conclusion
Our
experiments revealed that the presented setup of an MR scanner in front of a
horizontal ion beam line enables high quality MR imaging while simultaneously
irradiating a target using active beam scanning. One limitation of this study
is the small range of analysed MR pulse sequences that need to be adapted
towards a more realistic clinical application. Still, these experiments pave
the way for further studies on the road to MR-guided particle radiotherapy.
(1) Green OL et al. Med Phys. 2018;45(8)
(2) Hoffmann A et al. Radiat
Oncol. 2020;15(1)
(3) Schellhammer SM et al. Phys Med Biol.
2018;63(23)