3D Portal Dosimetry for extreme hypofraction: pre-treatment and in vivo verification
Mariana Pereira,
Portugal
PD-0805
Abstract
3D Portal Dosimetry for extreme hypofraction: pre-treatment and in vivo verification
Authors: Mariana Pereira1, Joep Stroom1, Ariana Rocha1, Carlo Greco1, Sebastiaan Nijsten2
1Champalimaud Centre for the Unknown, Department of Radiation Oncology, Lisbon, Portugal; 2Maastricht University Medical Centre, Department of Radiation Oncology (MAASTRO Clinic), Maastricht, The Netherlands
Show Affiliations
Hide Affiliations
Purpose or Objective
Standard
gamma-analysis for individual plan QA can be difficult to interpret and its
clinical relevance is doubtful. Deviations from clinical objectives are readily
understandable by DVH-based portal transit dosimetry. We aim to demonstrate the
feasibility of our in-house developed portal dosimetry solution for 3D
dosimetric verification of extreme hypofractionated VMAT treatments, using DVH
metrics.
Material and Methods
Energy fluency measured with an aS1200 Varian EPID is
used by our EPICoreMedPhys (ECMP) software to perform 3D dose verification without
(pre-treatment) and with a phantom (in phantom) or patient in the beam (in vivo). ECMP reconstructs 3D dose using a Monte Carlo code (XVMC) and compares
it to the 3D TPS dose (AAA, Eclipse v15.6). The in phantom tests were performed using the ArcCheck cylindrical
phantom. 30 hypofractionated
(Dfx>4Gy)
VMAT plans (13 Prostate,
5 Bone, 4 Brain, 4 Lymph Nodes, 2 Gyneacologic, 1 Pancreas and 1 Lung) with 6 and 10 FFF beams
were included. To study the influence of plan complexity, the mean distance
between opposing MLC leaves (mdMLC) was calculated for each plan and correlated
with median dose differences (ΔD50) between planned and reconstructed dose
distributions for the VOI defined by the planned 50% isodose volume (VID50).
For in vivo measurements, we selected
12 prostate plans (5×9Gy) treated with 10 FFF beams, yielding 32 fractions in vivo. Average DVH differences (ΔD2, ΔD50 and ΔD98) for the VID50, targets and OARs
were determined.
Results
Table 1.A shows our pre-treatment and in phantom results. Substantial dose
differences were found for mdMLC <1cm with highly modulated fields, yielding
an average ΔD50 for VID50 of -4.7
± 2.8% and -2.4 ± 1.7%, respectively, which can go (partly) unnoticed when
using gamma passing rates. Geometric and dosimetric calibration of these very
small leaf distances is complicated in many aspects (LINAC, dosimeters, TPS,
ECMP, etc.), so for further analysis we concentrated on 12 hypo-fractionated
prostate treatments with mdMLC of 1-2cm. Table 1.B shows smaller systematic
dose differences (<1%
underdose) for in phantom
prostate measurements, still explainable by minimal errors in leaf settings
like the dosimetric leaf gap (DLG). For in
vivo measurements, mean underdosage increases up to 5%, except for the
urethra wall that is enclosed by the PTV (Fig.1). Apart from tissue
inhomogeneities, anatomical changes and set-up errors that take effect during
patient’s treatments, we are currently investigating whether the extra
underdose might stem from residual uncertainties in the transit EPID dose
conversion itself and from differences in 3D dose calculation algorithms (XVMC
vs AAA).
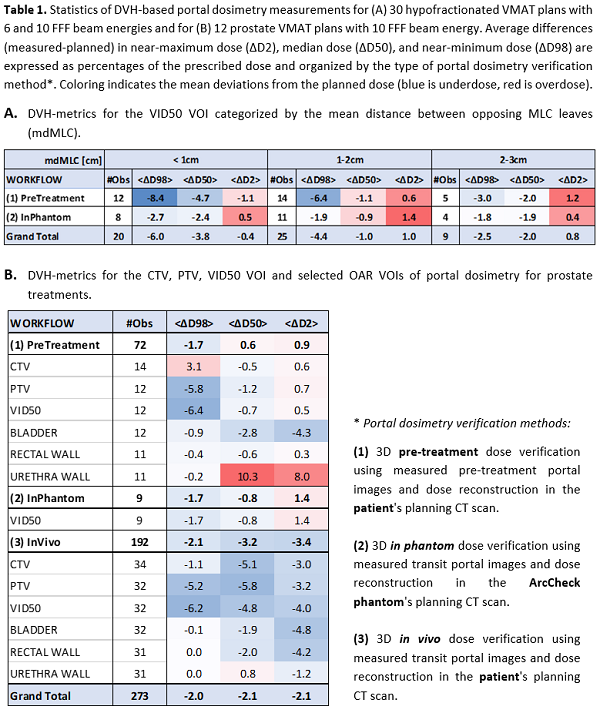
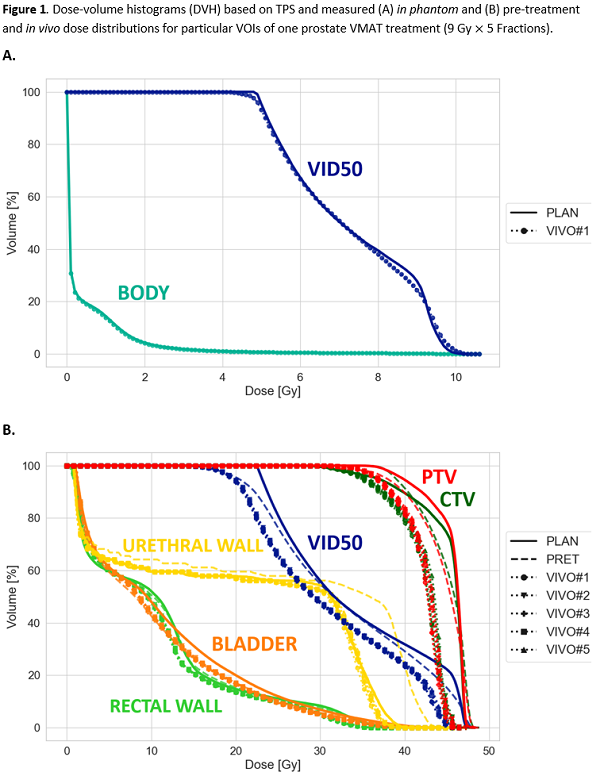
Conclusion
Our
portal dosimetry 3D dose deviations increase with decreasing leaf distances
used in complex RT plans. The very small segments can cause true underdosages
due to calibration inaccuracies but might also decrease the accuracy of the
portal dosimetry model that needs adjustment.