Improving spatial fidelity and image quality of mid-position MRI for lung radiotherapy
Katrinus Keijnemans,
The Netherlands
PD-0074
Abstract
Improving spatial fidelity and image quality of mid-position MRI for lung radiotherapy
Authors: Aart van Bochove1, Katrinus Keijnemans1, Pim Borman1, Astrid van Lier1, Martin Fast1
1University Medical Center Utrecht, Department of Radiotherapy, Utrecht, The Netherlands
Show Affiliations
Hide Affiliations
Purpose or Objective
Respiratory
motion is a large source of uncertainty for radiotherapy, which can be reduced
by treating in the time-weighted average/mid-position (midP) anatomy. A midP
image can be derived from a respiratory-sorted 4D-MRI, which usually has a poor
resolution. We propose combining a 4D-MRI with an additional, navigator-triggered scan
which can be acquired at high resolution, to maximize spatial fidelity and
image quality of the midP image. Here, we investigate the quality of improved
midP MRI imaging.
Material and Methods
We
previously developed a simultaneous multi-slice (SMS) accelerated coronal TSE
4D-MRI sequence. A 4D-midP image (2×2×2 mm3 voxel size) can be derived by deformably warping all 4D phases to the midP
anatomy, and calculating a time-weighted median (Fig 1a). Combining the 4D-MRI
with a navigator-triggered end-exhale axial PROPELLOR (MVXD) scan (Fig 1b),
yields a higher-resolution MVXD-midP image (0.5×0.5×3.5 mm3 voxel size). After warping the MVXD image to all 10 4D phases in ADMIRE v3.22.2 (Elekta
AB, Stockholm, SWE), the time-weighted average of the deformable vector fields
(DVFs) is used to warp the MVXD image to the midP.
Both
midP images were calculated for 13 patients and 2 healthy volunteers, scanned on
a 1.5T Ingenia MR-sim (Philips Healthcare, Best, NL).
The
consistency of the DVFs is quantified using the Distance Discordance Metric
(DDM) within the body. The midPs are validated by manual, translation-only
registrations of each 4D-phase and midP to end-exhale, based on a reference structure:
for 8 patients a tumor (9 tumors in total), and for 2 volunteers the liver-lung
interface (6 4D-MRI scans in total). Patient data was used if a clinical GTV
delineation was available, the tumor moved due to respiration, and the tumor
was visible in the 4D-MRI. The ground-truth translation from the midP to
end-exhale, based on all 4D phases, is compared to the translation from the calculated
midPs to end-exhale.
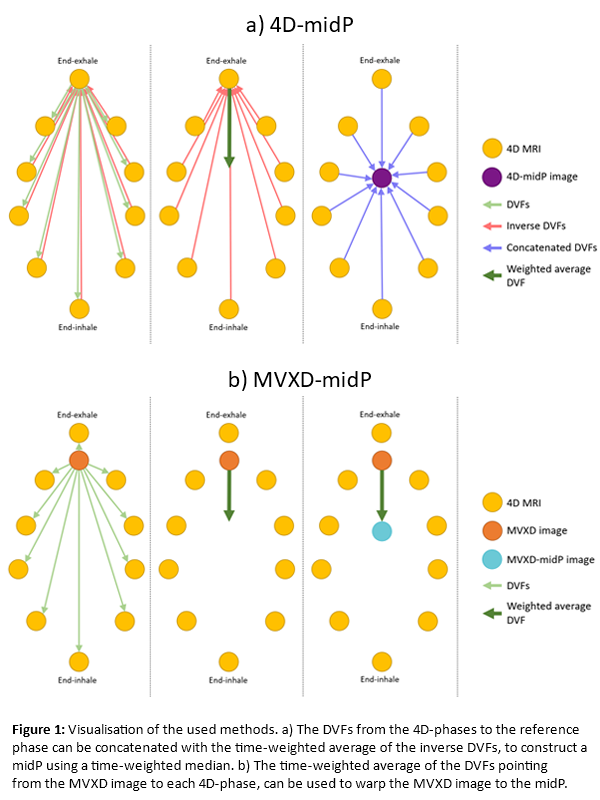
Results
Figure 2
shows midP images for an example patient. The MVXD-midP is much sharper than
the 4D midP.
Mean (highest
95%) DDM values of 0.9 (3.1) mm (4D-midP) and 1.5 (4.0) mm (MVXD-midP) were found
for patients, and 0.9 (3.1) mm and 1.5 (4.0) mm for volunteers. The percentage of
DDM values <2 mm was 88% (4D-midP) and 76% (MVXD-midP) for patients, and 87%
and 76% for volunteers. Elevated DDM values were found in areas with pulsatile
flow (heart, blood vessels) and ghosting (skin), as expected.
The
manual registrations show a mean (max) craniocaudal difference between the midP
and ground-truth translations of 0.3 (1.0) mm (4D-midP) and 0.5 (1.5) mm
(MVXD-midP) for patients, and 0.6 (1.4) mm and 0.8 (1.4) mm for volunteers.
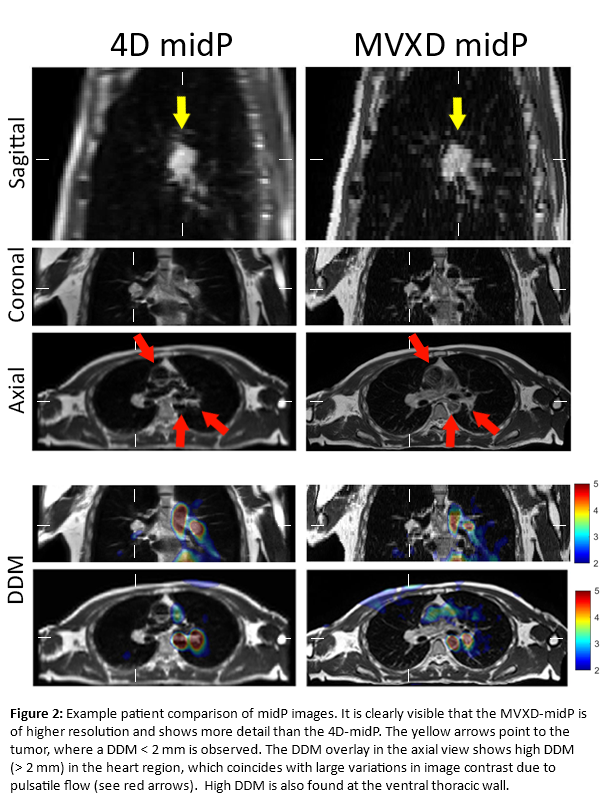
Conclusion
By
combining a navigator-triggered MVXD image with an SMS-4D-MRI image, we constructed
a dramatically sharper midP image. DDM analysis shows that the MVXD-midP is
slightly less reliable than the 4D-midP, but this has limited influence on the regions
of interest. Using this higher-resolution MVXD-midP could improve treatment planning.