Optimising tissue-equivalent materials for proton therapy
Hannah Cook,
United Kingdom
OC-0937
Abstract
Optimising tissue-equivalent materials for proton therapy
Authors: Hannah Cook1, Mikaël Simard2, Nathan Niemann3, Callum Gillies4, Hugo Palmans5, Mohammad Hussein6, Hugo Bouchard7, Gary Royle1, Ana Lourenço6
1University College London , Medical Physics and Biomedical Engineering Department , London , United Kingdom; 2University of Montreal , Department of Physics , Montreal , Canada; 3Bartholomew Hospital , Medical Physics Department , London , United Kingdom; 4University College London Hospital , Medical Physics Department , London , United Kingdom; 5MedAustron Ion Therapy Centre , Medical Physics Group , Wiener Neustadt, Austria; 6National Physical Laboratory , Medical Radiation Science , London , United Kingdom; 7University of Montreal , Department of Physics, Montreal , Canada
Show Affiliations
Hide Affiliations
Purpose or Objective
Phantoms are a vital tool for
both reference and end-to-end audits in radiotherapy. Phantom-based dosimetric audits
have been shown to improve confidence and consistency of radiotherapy treatments
at clinical centres. Recently, there has been an increase in the number of
proton therapy centres within the UK and worldwide. However, due to differences
in radiation interaction with matter between photon and proton beams,
previously used X-ray phantoms and phantom materials are suboptimal for proton
therapy. Research shows current tissue-equivalent materials cause large
uncertainties in proton therapy measurements. Consequently, there is a need for
photon and proton optimised tissue-equivalent materials.
Material and Methods
We have developed a mathematical
model using MATLAB which enables the formulation of tissue-equivalent
materials considering physical properties, photon interactions but also proton stopping
power, absorption, and scattering interactions. Using a non-linear optimisation
algorithm, this work has formulated new bone- and muscle-equivalent epoxy resin-based
materials in terms of their photon and proton interaction parameters. However, perfectly
matching all interactions is challenging, therefore, a weighted cost function
was defined according to the relative importance of each photon and proton
interaction. Mass density, mass attenuation and relative stopping power (RSP) were
assigned the highest weightings due to their impact on the materials ability to
be correctly characterised during the imaging and treatment planning process (TPS)
as well as providing accurate proton dosimetry measurements.
The formulated materials have been
manufactured and characterised via the use of single-and dual-energy CT as well
as proton water-equivalent thickness measurements at The National Physical
Laboratory (UK) and University College London Hospital. Monte Carlo simulations
(FLUKA) were completed to calculate the fluence correction factors of the
materials, which provides a more detailed understanding of nuclear interaction
equivalence of the material. Each material’s RSP was also compared to the
clinical TPS assigned RSP using the HU-RSP calibration curve to check their use
for end-to-end audit purposes.
Results
Table 1 show that the new optimised materials
score better than current commercial phantom materials when considering all-important physical properties, photon interactions, proton stopping power, absorption,
and scattering interactions.
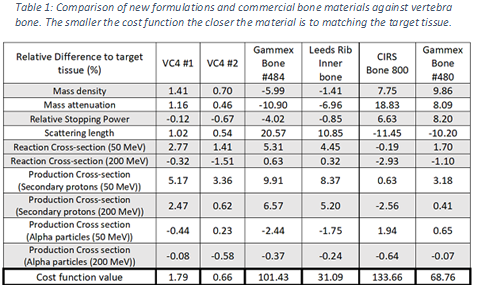
Experimental results suggest the new
bone and muscle formulations adequately mimic target tissue parameters such as
the mass density and RSP with acceptable differences of 1-3%.
Conclusion
Results suggest that the proposed bone
and muscle formulations can be used for the development of future photon and proton-optimised
dosimetric phantoms. This work highlights the potential of this new mathematical
model as a tool in the creation of phantoms with optimised tissue materials for
photon and proton beams.