Challenges in modelling the Agility multileaf collimator in TPSs and current needs for improvement
PD-0583
Abstract
Challenges in modelling the Agility multileaf collimator in TPSs and current needs for improvement
Authors: Victor Hernandez1, Agnes Angerud2, Evelien Bogaert3, Mohammad Hussein4, Matthieu Lemire5, Julia Garcia-Miguel6, Jordi Saez7
1Hospital Universitari Sant Joan de Reus, Medical Physics, Reus, Spain; 2RaySearch Laboratories AB, R&D, Stockholm, Sweden; 3Ghent University Hospital and Ghent University, Radiation Oncology, Ghent, Belgium; 4National Physical Laboratory, Metrology for Medical Physics Centre, Teddington, United Kingdom; 5CIUSSS de l'Est-de-l'Ile-de-Montreal, Medical Physics, Montreal, Canada; 6Consorci Sanitari de Terrassa, Radiation Oncology, Terrassa, Spain; 7Hospital Clinic de Barcelona, Radiation Oncology, Barcelona, Spain
Show Affiliations
Hide Affiliations
Purpose or Objective
The goals of this study were to investigate the challenges faced when modelling the Agility MLC in commercial TPSs, how they relate to the variability found in the MLC parameters used by the community, and to explore how the implemented MLC models could be improved in the future.
Material and Methods
Four Elekta linear accelerators from different centres, all equipped with the Agility MLC, were evaluated. Three centres used the RayStation TPS and one used Monaco. For comparison purposes, data from four Varian linear accelerators with the Millennium 120 MLC were also included. Average doses measured with asynchronous sweeping gap (aSG) tests (Fig 1a) were used to characterize and compare the characteristics of the Millennium and the Agility MLCs and to assess the MLC model in the TPSs. The FOURL test included in the ExpressQA package, provided by Elekta, was also used to evaluate the tongue-and-groove with radiochromic films. Finally, raytracing was used to investigate the impact of the MLC geometry and to understand the results obtained for each MLC.
Results
The geometry of the Agility produces dosimetric effects associated with the rounded leaf end up to a distance 20mm away from the leaf tip end measured at the isocenter plane. This affects the tongue-and-groove shadowing, which progressively increases along the distance to the tip end. This can be quantified by the average doses for the aSG tests and their decrease as a function of s (Fig 1b).
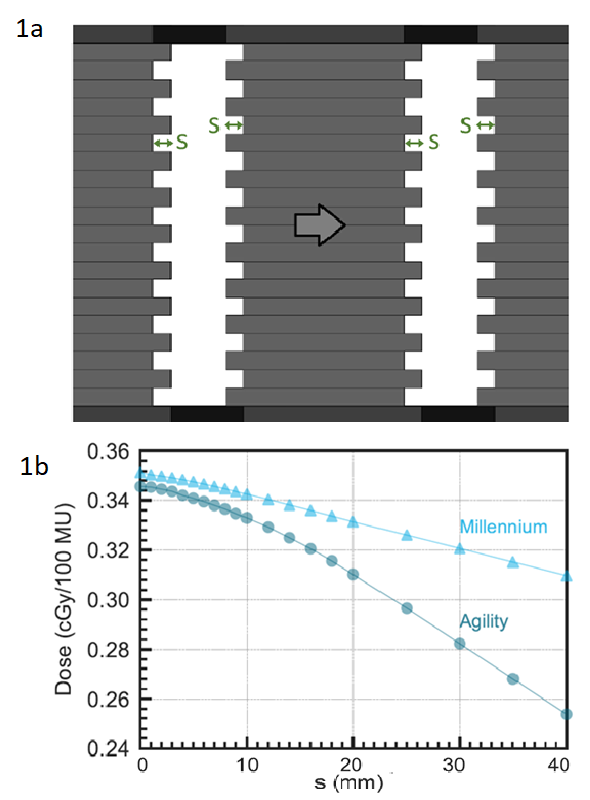
These doses can also be used to compute the fluence reduction at the leaf sides as a function of the distance to the leaf tip (s) (Fig 2a) as well as its first derivative, which is proportional to the effective tongue-and-groove width (Fig 2b). RayStation and Monaco did not account for this effect, which made trade-offs in the MLC parameters necessary and greatly varied the final MLC parameters used by different centres (Fig 2c).
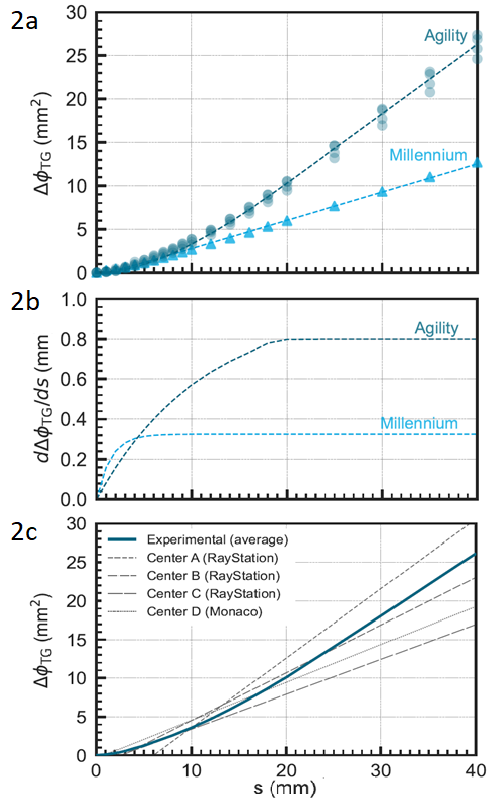
The parameters used by each centre strongly depended on the procedure used for its determination (e.g., FOURL test vs tuning with clinical plans). In comparison, these leaf tip effects were much less pronounced for the Millennium MLC. Raytracing showed that these challenging effects were directly related to the MLC geometry and that the characteristics responsible for the large leaf tip effects of the Agility were its tilting design and its small source-to-collimator distance.
Conclusion
The MLC models implemented in RayStation and Monaco could not accurately reproduce the leaf tip effects for the Agility. Therefore, trade-offs are needed and the optimal MLC parameters are dependent on the specific characteristics of treatment plans. Refining the MLC models for the Agility to better approximate the measured leaf tip and tongue-and-groove effects is needed to extend the validity of the MLC model, reduce the variability in the MLC parameters used by the community, and facilitate the standardization of the MLC configuration process.
[1] Phys Med Biol. 2017 Aug 1;62(16):6688-6707
[2] Phys Med Biol. 2020 Jul 27;65(15):155006