Design and assembly of a non-invasive radiation detector to measure the AIF in dynamic PET.
PD-0586
Abstract
Design and assembly of a non-invasive radiation detector to measure the AIF in dynamic PET.
Authors: Liam Carroll1, Youstina Daoud2, Shirin A. Enger3,2
1McGill University, Biomedical Engineering, Montreal, Canada; 2Jewish General Hospital, Lady Davis Institute, Montreal, Canada; 3McGill University , Oncology, Medical Physics Unit, Montreal, Canada
Show Affiliations
Hide Affiliations
Purpose or Objective
The presence of hypoxic regions in tumor volumes reduces the effectiveness of common treatment techniques including radiotherapy, chemotherapy and immunotherapy. It is possible to map these hypoxic volumes using F-18-fluoromisonidazole (FMISO) dynamic positron emission tomography (dPET). Tumor hypoxia can then be used to predict response to therapy, perform radiotherapy dose escalation and other treatment optimizations. To perform dPET, the time-course activity concentration in the patient’s arteries, called the arterial input function (AIF), must be measured. The gold-standard method to measure the AIF is through arterial cannulation to sample arterial blood throughout the dPET scan. This process imposes a large burden on centres who wish to perform dPET scans. Our lab is developing a non-invasive radiation detector (NID) designed to measure the AIF, removing the need for arterial blood sampling. This work presents the design and fabrication of the NID.
Material and Methods
The sensitive volume of the detector consists of 64 plastic scintillating fibers, 10 cm in length, arranged in 2 layers of 32 fibers. Serial polishing of one fiber-end was performed for each fiber to prepare them to be coupled to 16 cm long optical transmission fibers. The coupling was performed by placing the two fiber-ends in a transparent heat shrink tube filled with a UV curing optical epoxy. A 3D printed plastic shell with 20% infill was used to hold the fibers in the desired shape and block ambient light. The shell was also used to hold the bare end of the optical fibers in an 8x8 grid to allow them to be coupled to an 8x8 silicon photomultiplier (SiPM) array. An index matching silica sheet was placed between the SiPM array and the optical fibers. Velcro straps are used to hold a patient’s arm in place. Black acrylic sheets are used to block ambient light from reaching the scintillating fibers. The SiPM is connected to an electronic board to read-out the signal captured by the scintillating fibers. To test the NID functionality, an un-calibrated 18F solution was placed in the detector volume for a ten-minute acquisition. F-18 half-life was calculated and compared to literature values.
Results
Figure 1 illustrates the finished NID and accessories. The ergonomic design of the NID allows the patient to rest their arm on the detector surface without straining. This is important for longer dPET scan times to avoid patient movement. Figure 2 shows the measured decay of F-18 in the NID. The calculated half-life is within 4.5% of literature values. The same scan shows that 9 out of the 64 channels are non-functional.
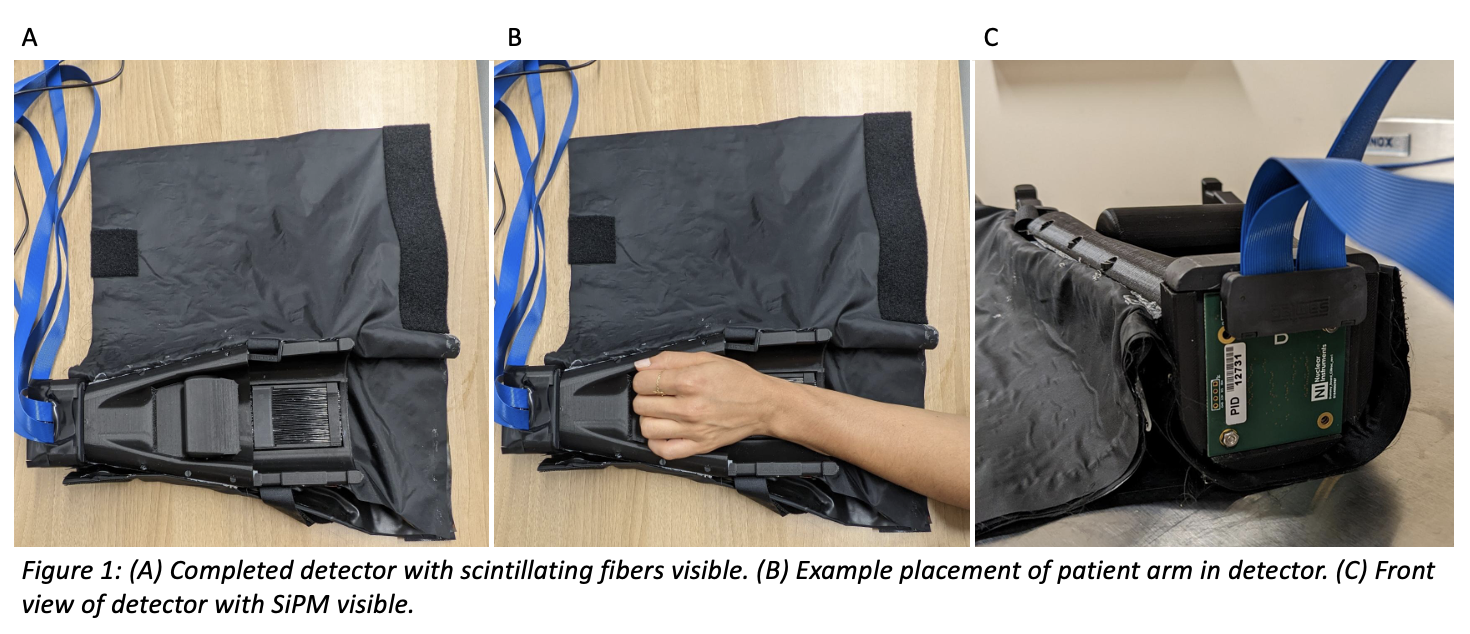
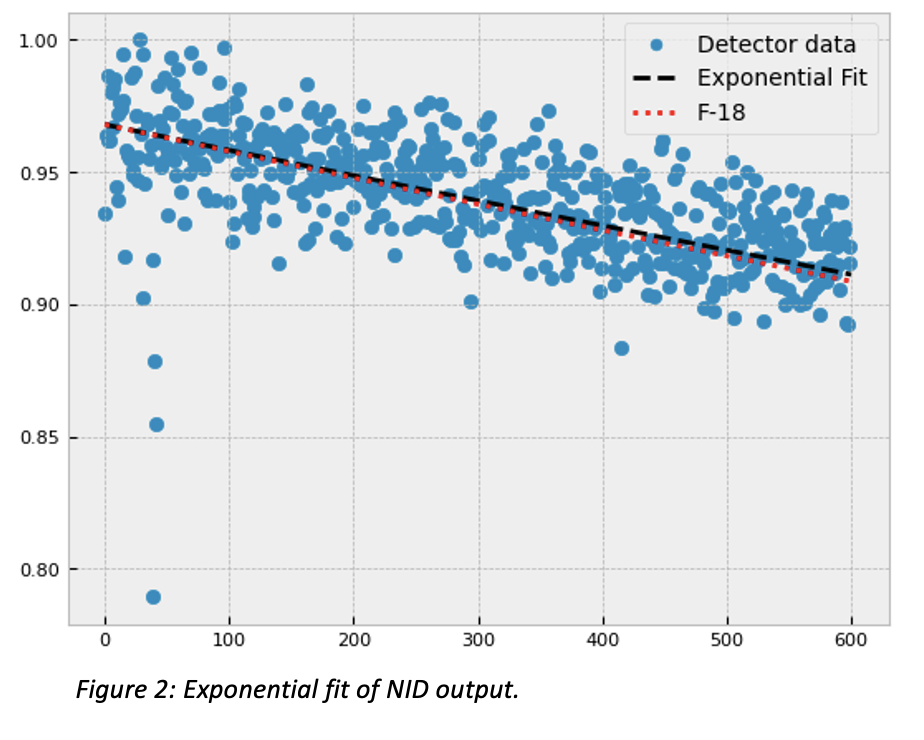
Conclusion
The constructed NID is functional and capable of measuring radiation from an F-18 source. These tests were done with F-18, but the NID would work with any PET radioisotope. The fiber-coupling procedure works, but further quality control steps are needed to remove non-functional channels. Additional measurements with clinical phantoms and patients are planned to characterise the NID.