Implementation of the DirectDensity CT reconstruction kernel for radiotherapy planning
Spyros Manolopoulos,
United Kingdom
PO-1856
Abstract
Implementation of the DirectDensity CT reconstruction kernel for radiotherapy planning
Authors: Rachael Tulip1, Spyros Manolopoulos1, Neil Richmond2, Christopher Walker2
1Northern Centre for Cancer Care - North Cumbria - Newcastle upon Tyne Hospitals NHS Foundation Trust, Radiotherapy Physics, Carlisle, United Kingdom; 2Northern Centre for Cancer Care - Newcastle upon Tyne Hospitals NHS Foundation Trust, Radiotherapy Physics, Newcastle-Upon-Tyne, United Kingdom
Show Affiliations
Hide Affiliations
Purpose or Objective
The Hounsfeld number–density (HN-D) calibration curve used in planning systems is energy dependent and discontinuous at soft tissue-like densities. Scanning patients at a kVp optimal for image quality requires multiple HN-D curves; increasing quality assurance burdens and adding potential confusion in planning processes. Traditionally HN-D curves are limited to one kVp resulting in suboptimal image quality for individual patients. Implementation of the DirectDensityTM (Siemens Healthineers GmBh Germany) reconstruction remedies this by generating one HN-D curve independent of kVp providing increased robustness to energy changes and allowing kVp optimisation. This work reports on linearity and energy change robustness investigations using DirectDensityTM.
Material and Methods
HN–D calibration curves at all available kVp were generated using an Advanced Electron Density Phantom (Sun Nuclear Corporation, WI, USA) on a SOMATOM go.Open Pro (Siemens Healthcare GmbH, Germany) CT scanner. The curves were validated in the RayStation treatment planning system (RaySearch Laboratories, Sweden) using a Gammex RMI467 phantom.
The kVp dependence of the Hounsfield Numbers (HN), for the Sm40 reconstruction, was investigated for 7 densities; 0.299 g/cc (Lung LN-300) to 1.925 g/cc (Cortical Bone).
A representative mediastinal VMAT plan was created using the CIRS Model 002LFC IMRT Thorax Phantom (SunNuclear, USA). Dose was calculated using the Qr40 (at 120kVp) and the Sm40 HN-D calibration curves at the kVp stated above. CT patient scans (corresponding to pelvis, thorax, Head and Neck and Brain) acquired at 120kVp (Qr40), were reconstructed with the Sm40 kernel. Their clinical plan was recalculated for dose comparison.
Results
DirectDensityTM produced linear HN-D curves with reduced energy dependence.
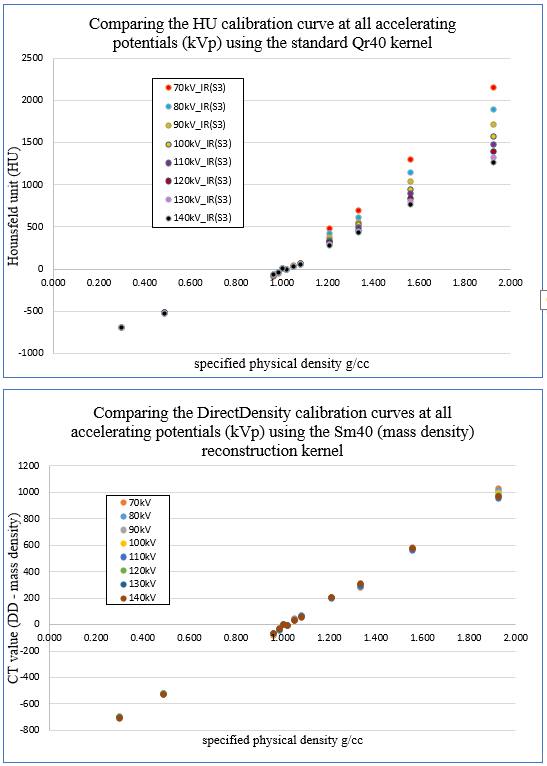
Fig1: kVp dependence of the HU number/CT value using Qr40 and Sm40.
For adipose, the mean HN (Sm40) =-70.8 (s.d. 7.2), equating to a density of 0.929g/cc (reference=0.960g/cc). For LN-300 the mean HN was -696.2 (s.d. 5.3) equating to a density of 0.304g/cc (reference=0.299g/cc). For cortical bone, the mean HN =983.9 (s.d. 28.8). This equates to a density of 1.984g/cc (reference=1.925g/cc). For bone inserts, an incidental finding where HN values decreased was observed at 110kVp
Fig2: HN with kVp for adipose and cortical bone
Dose distributions on the CIRS Phantom using Qr40 or Sm40 at different kVp showed the following mean differences for the PTV: D99% of -0.45% (s.d = 0.05), D50% of -0.07% (s.d. = 0.03) and D2% of -0.13% (s.d. = 0.06). Sm40 doses were lower than the Qr40 doses.
Dose comparisons between Sm40 and Qr40 for the patient scans showed differences for the PTV; D99% mean = -0.30% (s.d.=0.48), D50% mean=-0.35% (s.d.=0.40) and D2% mean=-0.28% (s.d. 0.46). Doses for Sm40 were lower than Qr40 except for the brain scans.
Conclusion
DirectDensityTM is found to be clinically acceptable for radiotherapy treatments. The HN-D curve is more linear and less energy dependent compared to the standard method.