Study of radial waveguide design for a compact dielectric wall accelerator
PO-1833
Abstract
Study of radial waveguide design for a compact dielectric wall accelerator
Authors: Morgan Maher1, Christopher M. Lund1, Julien Bancheri1, David G. Cooke2, Jan Seuntjens1,3
1McGill University, Medical Physics Unit, Montreal, Canada; 2McGill University, Department of Physics, Montreal, Canada; 3University of Toronto, Princess Margaret Cancer Centre, Radiation Medicine Program, Toronto, Canada
Show Affiliations
Hide Affiliations
Purpose or Objective
To computationally model electromagnetic distortion in radial parallel plate waveguides. This work is part of an effort to refine the design parameters of the electromagnetic field delivery modules for a compact dielectric wall accelerator for proton radiotherapy. The field delivery modules, composed of high-voltage pulse-generating circuits and parallel plate waveguides, are stacked along the beam axis and sequentially deliver short-lived (∼1 ns), high-gradient (∼100 MV/m) electric field pulses as the particle bunch transits the device. The high-gradient electric fields promise a compact accelerator (∼3 m), but pose design challenges, especially in the context of electrical breakdown. The proposed radial geometry offers a passive field amplification, which alleviates the electrical stress on upstream circuitry. However, temporal distortions of the waveform also occur, which can lead to undesirable characteristics in the accelerating field (mainly related to longitudinal stability of the particle bunch). Understanding the impact that waveguide geometry and composition have on the distortions is crucial to selecting a design that is both feasible to construct and suitable for the acceleration of a clinical proton beam.
Material and Methods
COMSOL Multiphysics (2D Axisymmetric, Transient Electromagnetic Waves) was used to model radial parallel plate waveguides. The waveguide was uniformly excited at the outer radius with a Gaussian voltage pulse (f ), and the output pulse (h) at the inner radius was measured (Fig 1). The frequency response of the system (G, i.e., frequency-dependent amplification factor), was calculated numerically (Eq 1) and studied for various geometric (inner radius rin , outer radius rout , plate separation d) and material (relative permittivity εr ) parameters (Fig 1).
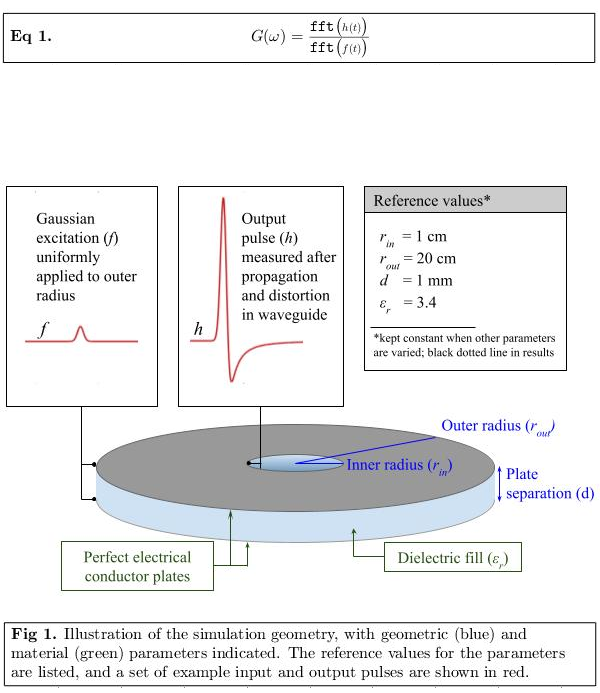
Results
All simulated geometries exhibited non-uniform amplification across the bandwidth of f , with amplification factors approaching a plateau at higher frequencies (Fig 2). Decreasing rin , increasing rout, and increasing εr all increased amplification of the signal, although the frequency-dependence of this effect was unique to each parameter. Additional study is needed to understand the interplay between the parameters, and to further quantify the frequency-dependence of the responses. Changing d had no effect on G.
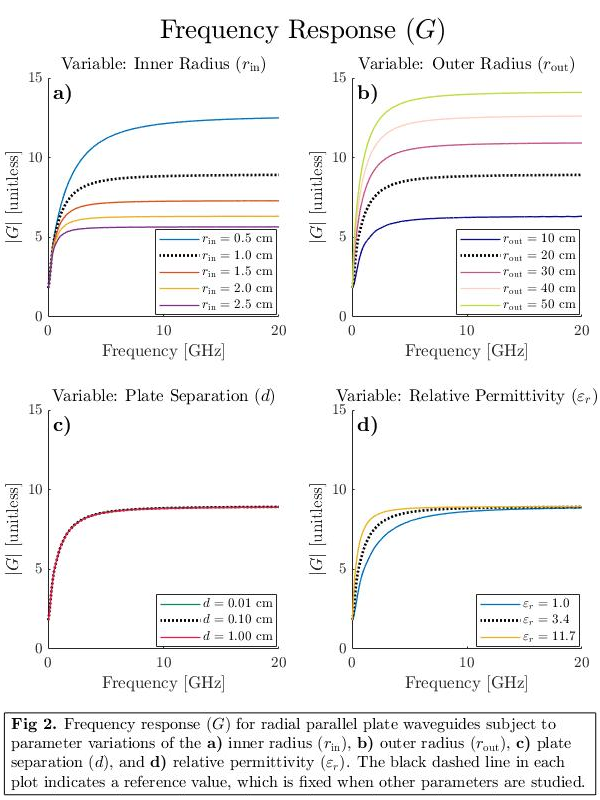
Conclusion
Decreasing the inner radius, increasing the outer radius, and increasing the relative permittivity of the waveguide all led to increased amplification across the frequencies studied. This information can be used in conjunction with external factors in the accelerator design (particle bunch stability, material cost/availability, size/weight of the device, etc.) to select an appropriate waveguide geometry and composition. These results also inform the power output and pulse shaping requirements of the upstream circuit design as they provide an important bridge between the strength and temporal profile of the injected pulses and those of the accelerating fields.