Together we are strong: combining DNA repair and immune checkpoint inhibitors to enhance radiosensitisation
The exploitation of a defect in the DNA damage response (DDR) in tumour cells to optimise therapeutic response has been used successfully in tumour therapy. After immune checkpoint inhibition or blockade therapy (ICI or ICB) was approved for the treatment of tumours with defective repair mechanisms for DNA mismatches, interest in the interplay between ICI and inhibitors of the DDR for combinational use with radiotherapy has been increasing. This treatment may represent an attractive option to complement existing therapies. Currently, the combined triple approach is in the preclinical setting and has shown initial promising results.
The most mature treatment of this kind is the combined therapy with inhibition of poly(adenosine diphosphate (ADP)-ribose) polymerase 1 (PARP1). Mutations in the BRCA1 and BRCA2 genes have been associated with increased infiltration of T lymphocytes and enhanced expression of programmed death-ligand 1 (PD-L1) in response to activation of the cyclic guanosine monophosphate-adenosine monophosphate (GMP-AMP) synthase (cGAS) pathway that stimulates interferon genes (the STING pathway) [1]. It has been found that PARP1 inhibition alone activates the cGAS/STING pathway and that this leads to further enhancement of therapeutic effects when PARP1 inhibition is combined with ICI [2]. Upregulation of PD-L1 expression is reported to be dependent on the levels of the kinases ataxia telangiectasia mutated (ATM), ataxia telangiectasia and RAD3-related (ATR) and checkpoint kinase 1 (CHK1), which appear to be responsible for the observed effects [3]. Consequently, inhibitors of this signalling cascade represent further approaches for the combined treatment of DDR and ICI in the preclinical setting. ATR inhibition has been shown to attenuate radiotherapy-induced CD8+ T-cell depletion and increased CD8+ T-cell activity in combination with conformal radiotherapy. Mechanistically, ATR inhibition appears to block radiation-induced PD-L1 upregulation in tumour cells, thereby reducing the number of tumour-infiltrating regulatory T cells (Tregs) [4].
Other findings support these observations. Inhibitors of ATR have been found to enhance significantly the innate immune response that is triggered by radiotherapy. The cGAS/STING-dependent DNA sensing pathway is responsible for radiotherapy-induced type I interferon (IFN) signalling. This is triggered by the presence of DNA fragments that are released due to DNA damage [5].
ATR inhibition in combination with fractionated radiotherapy has also been found to enhance tumour inhibitory effects in cases of human papillomavirus (HPV)-related malignancies. In these cases, significant radiosensitisation by ATR inhibition has been observed, and this radiosensitisation is accompanied by a marked increase in DNA damage and immune cell infiltration. ATR inhibition plus doses of aximiza radiation (IR) have been reported to cause gene expression that shows a signature consistent with the observed type I/II IFN response. In vivo, significant modulation of cytokine gene expression has been observed [6].
In an HPV-negative mouse model of oral squamous cell carcinoma, it has been observed that inhibition of ATR enhances the level of radiotherapy-induced inflammation of the tumour microenvironment, and that natural killer cells play central roles in the aximization of treatment efficacy [7]. The use of radiotherapy and ICI has also been found to cause an immunostimulatory effect of ATR inhibition in hepatocellular carcinoma. This occurs because ATR inhibition with radiotherapy increases rates of CD8+ T-cell infiltration and reverses the immunosuppressive effect of radiotherapy on the number of Tregs [8].
It has been shown that to inhibit ATM, activation of the innate immune response through enhanced induction of DNA damage increases the efficacy of ICI. It has previously been shown that inhibition of ATM alone increases rates of expression of tumoural type-I IFN, independently of the cGAS/STING pathway. The combination of ATM inhibition and radiotherapy increases these rates even more and, accordingly, leads to increased levels of IFN production and antigen presentation. Furthermore, the silencing of ATM increases rates of expression of PD-L1 and enhances the sensitivity of pancreatic tumours to antibodies that block PD-L1 [9]. Inhibition of the DNA-dependent protein kinase catalytic subunit (DNA-PKcs), which is a DDR sensor that is responsible for DNA repair via non-homologous end-joining, has also been shown to have an immunomodulatory effect. The combination of doses of IR and DNA-PKcs inhibition enhances the rates of production of cytosolic double-strand DNA and rates of signalling by tumour-associated type-I IFN, independent of the actions of cGAS and STING. In parallel, PD-L1 expression has been reported to be stimulated and the simultaneous use of anti-PD-L1 in combination with IR and DNA-PKcs inhibitors enhances anti-tumour immunity [10].
The cell cycle kinase known as WEE1 is another interesting DDR protein that has been found to stimulate the immune response in combination with doses of IR. In several models, it has been observed that inhibition of WEE1 leads to the accumulation of DNA damage and that combination treatment improves tumour control. The combination of radiotherapy and WEE1 inhibition has not only increased tumour-specific cytotoxicity, but also improved susceptibility to the killing of tumour cells by T lymphocytes and their response to PD-axis ICI [11].
The observations listed above suggest that the combination of recently tested inhibitors of DDR and immune response in preclinical models opens further options for radiation sensitisation and represents attractive opportunities for the use of such combinations in tumour therapy.
Sandra Classen and Kerstin Borgmann
Laboratory of Radiobiology & Experimental Radiooncology
Department of Radiotherapy and Radiation Oncology
Center of Oncology
University Hospital Hamburg-Eppendorf
Hamburg, Germany
borgmann@uke.de
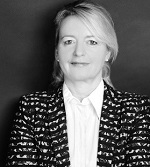
Kerstin Borgmann
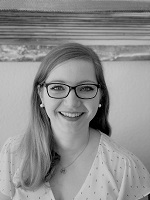
Sandra Classen
References
- Parkes, E.E., et al. Activation of STING-Dependent Innate Immune Signaling By S-Phase-Specific DNA Damage in Breast Cancer. J Natl Cancer Inst, 2017. 109(1).
- Shen, J., et al. PARPi Triggers the STING-Dependent Immune Response and Enhances the Therapeutic Efficacy of Immune Checkpoint Blockade Independent of BRCAness. Cancer Res, 2019. 79(2): p. 311-319.
- Sato, H., et al. DNA double-strand break repair pathway regulates PD-L1 expression in cancer cells. Nat Commun, 2017. 8(1): p. 1751.
- Vendetti, F.P., et al. ATR kinase inhibitor AZD6738 potentiates CD8+ T cell-dependent antitumor activity following radiation. J Clin Invest, 2018. 128(9): p. 3926-3940.
- Feng, X., et al. ATR inhibition potentiates ionizing radiation-induced interferon response via cytosolic nucleic acid-sensing pathways. EMBO J, 2020. 39(14): p. e104036.
- Dillon, M.T., et al. ATR Inhibition Potentiates the Radiation-induced Inflammatory Tumor Microenvironment. Clin Cancer Res, 2019. 25(11): p. 3392-3403.
- Patin, E.C., et al. Harnessing radiotherapy-induced NK-cell activity by combining DNA damage-response inhibition and immune checkpoint blockade. J Immunother Cancer, 2022. 10(3).
- Sheng, H., et al. ATR inhibitor AZD6738 enhances the antitumor activity of radiotherapy and immune checkpoint inhibitors by potentiating the tumor immune microenvironment in hepatocellular carcinoma. J Immunother Cancer, 2020. 8(1).
- Zhang, Q., et al. Inhibition of ATM Increases Interferon Signaling and Sensitizes Pancreatic Cancer to Immune Checkpoint Blockade Therapy. Cancer Res, 2019. 79(15): p. 3940-3951.
- Xue, Z., et al. PD-L1 deficiency sensitizes tumor cells to DNA-PK inhibition and enhances cGAS-STING activation. Am J Cancer Res, 2022. 12(5): p. 2363-2375.
- Patel, P., et al. Enhancing direct cytotoxicity and response to immune checkpoint blockade following ionizing radiation with Wee1 kinase inhibition. Oncoimmunology, 2019. 8(11): p. e1638207.